This is the first part of three about aerodynamics in cycling. Cycling aerodynamics can be a confusing and mysterious topic for many cyclists. It shouldn’t be. Whether you’re a seasoned professional or a beginner, understanding aerodynamics will help you ride faster, smarter and make the best equipment choices. In fact, 70 to 90 percent of a cyclist’s effort is dedicated to overcoming air resistance when not climbing steep hills.
The present article aims to explain aerodynamics in cycling as a physical phenomenon. You will learn how much power is needed to double your speed (eight times more!), what aerodynamics drag is (yes, it is drag!) and why certain shapes are more aerodynamic than others. Once you truly understand these basics, you can already infer many things.
Part 2 will focus on how challenging it can be to assess the aerodynamics of bikes and components in the wind tunnel or real world and finally, part 3 will look at practical considerations. Together, all three articles should already make you a faster cyclist, as they will guide your riding and equipment choices. In future articles, we will often refer back to this trilogy when we discuss the specifics of particular cycling gear.
Aerodynamics does matter in cycling
Cycling is a simple affair, the power the rider puts in the pedal is translated into forward motion. What makes the difference between going fast and even faster is, on the one hand, the power available to the rider and, on the other hand, how to best overcome the forces of nature that work against the effort.
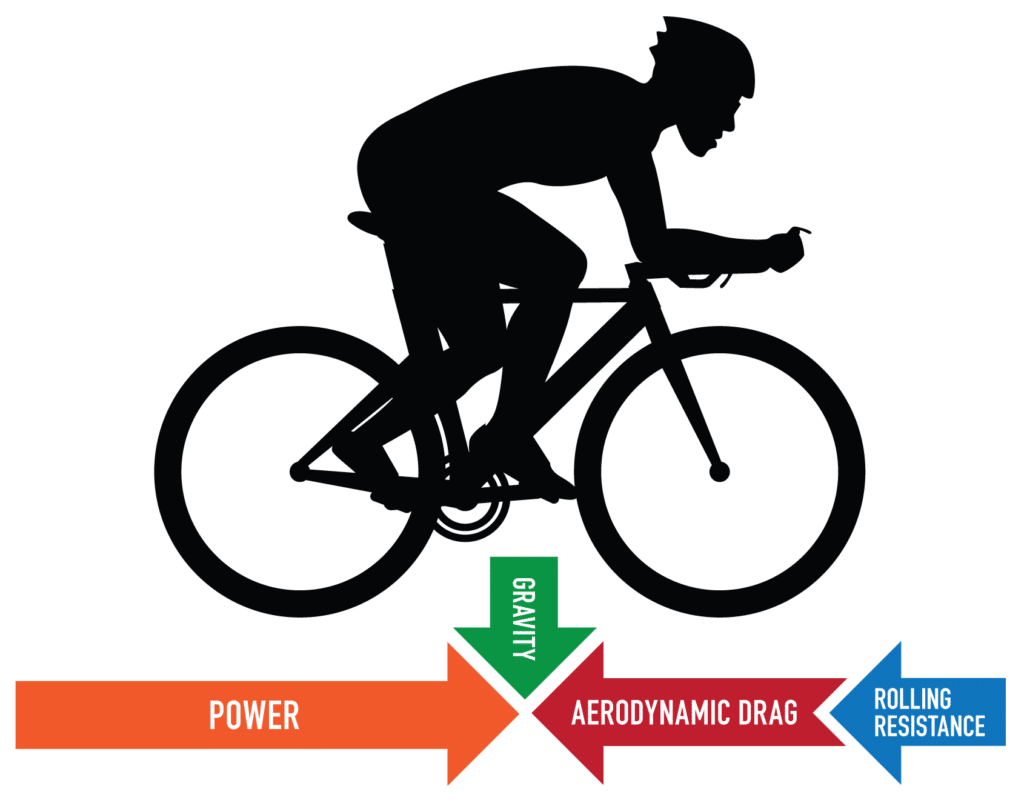
The illustration above shows the different forces at play. The power moves the riders forward, gravity pulls them into the ground (just imagine the difference between sliding an empty versus a full water bottle over the table), and then rolling resistance and especially aerodynamics work directly against power. To obtain the highest possible speed from the power we possess, we must reduce the impact of counter forces on our movement. We can achieve this by comprehending the dynamics and adapting ourselves and our equipment accordingly.
To maximize your cycling power, minimize the impact of counterforces such as gravity, aerodynamic drag, and rolling resistance. Understanding these factors can help you choose the right equipment.
As you will learn in the following paragraphs, aerodynamic drag is one of the most important forces to overcome, accounting for 70% to 90% of the counterforce. However, as we will also discover, it’s complex. But let’s start with the physics and theory first.
The laws of physics illustrate the importance of aerodynamics in cycling
When moving forward, the rider and bike have to overcome the air resistance or, more precisely, aerodynamic drag as they push the air in front of them out of the way. Below is the physics formula of this drag:
F_D = CdA \times \frac{1}{2}\rho \times v^2
- CdA (Coefficient of drag Area): In simple terms, it refers to how aero a system is. “Cd” describes how aerodynamic an object is and “A” refers to the size of the frontal area that “sees” the wind. It makes intuitive sense. A smaller object needs to push less air away and, therefore, experiences less drag. Similarly, a more aerodynamic shape goes with less drag through the air. Typical values for cyclists range from standard 0.5 to very aero 0.2
- ρ (greek letter rho): The density of the air. The less dense, the lower the drag. ρ is influenced by location and weather conditions. Air density is affected by atmospheric pressure, temperature, and humidity. At higher altitudes, air density decreases, which is why world record attempts were made at the Mexico City velodrome at 2230 Hm. As temperature increases, air density decreases. Similarly, the more humid it is, the less dense the air becomes.
- v: velocity, the speed at which the system travels. It’s cubed! Aerodynamic drag increases exponentially the faster you go. That’s one of the reasons why aero is such an essential factor.
To double your speed, you need 8x the power
The fact that aerodynamic drag increases exponentially, is why aero plays such a crucial role. Our human brains are inherently unable to grasp the effects of exponential systems, be it interest rates or, most recently, infection rates of COVID-19. In our context, it is very simple: to double your speed on the bike, you will need eight times the power to overcome the increase in aerodynamic drag.
Aerodynamic drag significantly affects cycling speed. When doubling your speed, you experience quadruple the drag and require eight times the power. Why? Because you hit twice as many air particles with twice the force.
Yes, 8 times! Why? If you want to travel at double the speed, you will hit twice the amount of air particles and hit them twice as hard. That’s already a 4 times increase in aerodynamic drag as described in the formula above. The power needed to overcome this drag is multiplied by velocity, which is double, as said.
Confusing? Here is a simplified formula:
\frac{new\space speed^3}{current\space speed^3} \times current\space power = new\space power
Assume your initial speed is 20 km/h, and you need 50 Watts to achieve it. If you aim to double it to 40 km/h, you will need 400 watts. Likewise, going from 30 km/h at 200 Watts to 40 km/h asks for about 474 watts. If you have a power meter and are familiar with these numbers, you may have already experienced how much more power you need to increase speed at high velocities compared to slower speeds.
It is called aerodynamic drag for a reason, it is just that
The simplified mental model for aerodynamics in cycling is the power needed to overcome the air resistance in front of us while moving forward. It is a good model, yet the reality is a little more complicated than that and fails to fully explain some of the effects we are seeing. Therefore, we must understand aerodynamic drag in more detail to understand why some shapes are more “aero” than others. Specifically, how the drag coefficient, or “Cd” in the formula above, can be influenced. There are two elements: pressure drag and friction drag.
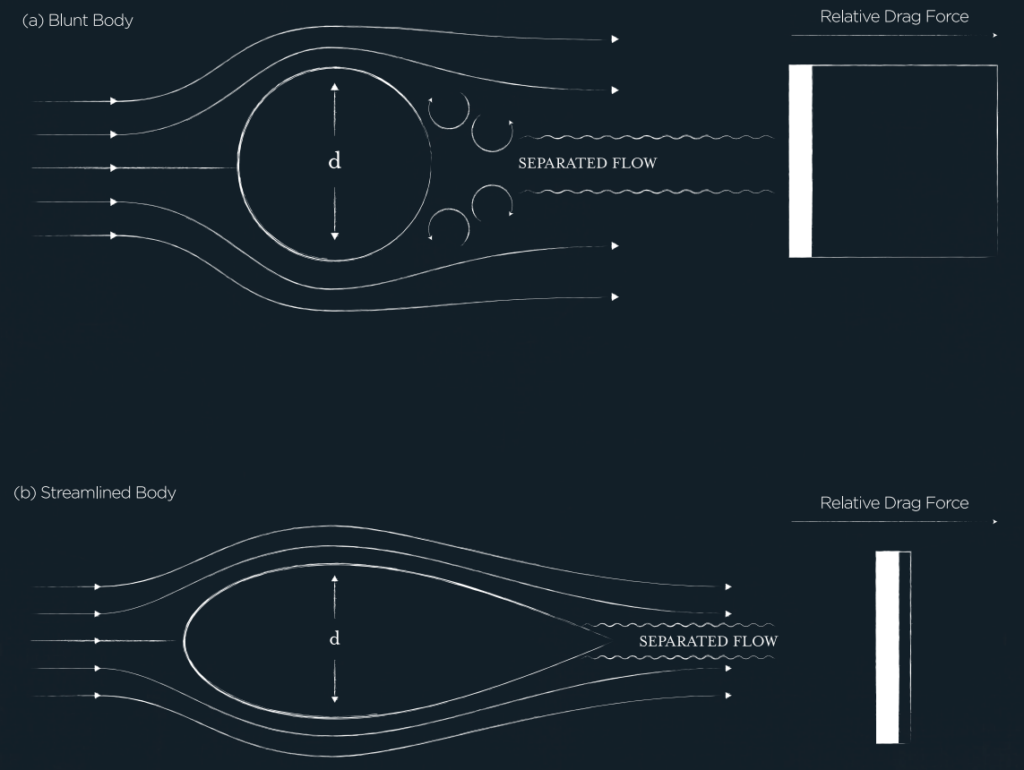
As we ride our bikes, the air in front of us becomes more compressed and is under high pressure, while the air behind us has less pressure. This pressure difference creates a force that slows us down, known as “pressure drag.” If an object is blunt, the airflow around it separates, causing a low-pressure space behind it. This results in more pressure drag, making the object less aerodynamic as it is “pulled from behind.” On the other hand, if an object is streamlined or “aero,” the air can flow around it without separating. This means that the low-pressure area is smaller, resulting in reduced pressure drag and, consequently, a more “aero” shape.
Aerodynamic drag is not simply the resistance of the air in front of you. It is a result of pressure differences between the front and back, as well as friction drag. Essentially, the low-pressure air behind you pulls you backward.
Furthermore, there is “friction drag,” which is the energy lost due to the friction of the air passing over the object. If the air sticks more to the object and flows less easily, more power is needed to move forward. Different materials and surface shapes can reduce the friction drag and, thus, make an object more aero.
Round is not a very good aero shape, be it steel tubes, shifting cables or legs
Based on what we have learned above, designing the optimal shape is much of an engineering challenge when designing aerodynamic bikes and components. Obviously, it is an optimization problem because aero is not the only criterium as the component must, most importantly, fulfill its function reliably, whether it is a head tube, seat post or helmet.
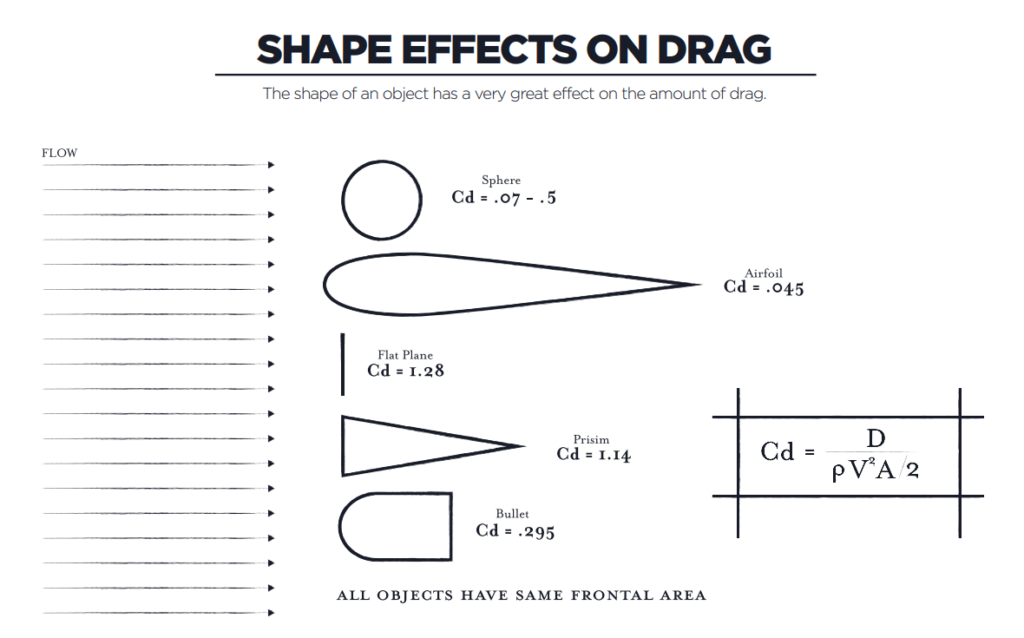
Much research by NASA and others has gone into researching the ideal aerodynamic shapes. Some examples are shown above. An airfoil shape’s drag coefficient (Cd) is 0.045 compared to 0.3 for a bullet and 0.5 for a sphere, assuming all have the same frontal area. It is difficult to grasp intuitively that a much bigger shape can have similar aerodynamic drag if it is a more aero. Nevertheless, the data shows there is a 2 to 10 times difference in Cd between the round lugs of an old steel bike and the modern aero-optimized frame shapes. That’s quite a lot! On the extreme, that 5 mm tiny, round shifting cable hanging from your bike might cause the same aerodynamic resistance as the 5 cm wide aero-optimized headtube.
That tiny 5mm, round shifting cable hanging from your bike may cause the same aerodynamic resistance as the 5cm wide aero-optimized headtube.
These differences are most pronounced at the front of the bike, where the rider and bike hit clean air, in comparison to the back part of the bike. Therefore, front wheels, handlebars, internal cable routing and helmets are all critical areas for aerodynamic optimization, as we will discover in more detail in future articles.
The three ways to be aero: be smaller, more streamlined and more slippery
In summary, to minimize the CdA part of the aerodynamic drag, you want to have the minimum frontal area in the wind, the « A » part of the equation. Furthermore, have a slippery surface to the air and be of streamlined shape for the « Cd » part. The engineers of the bike industry try to optimize all these aspects in the modern design of bike frames, wheel design, helmets, clothing and all other components. The question remains: who does the best job? Which equipment is indeed the most aero for my intended use?
Well…it depends. As we will learn in part 2 of this series on aerodynamics in cycling, assessing the truly most aerodynamic system is highly complex despite computational fluid dynamics (CFD), wind tunnels and the latest real-world testing technology.
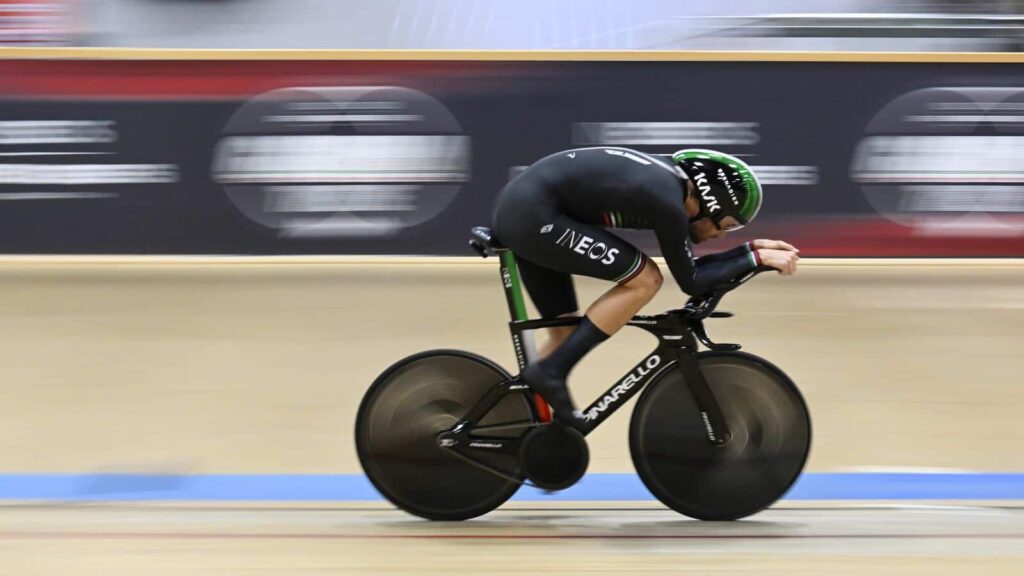
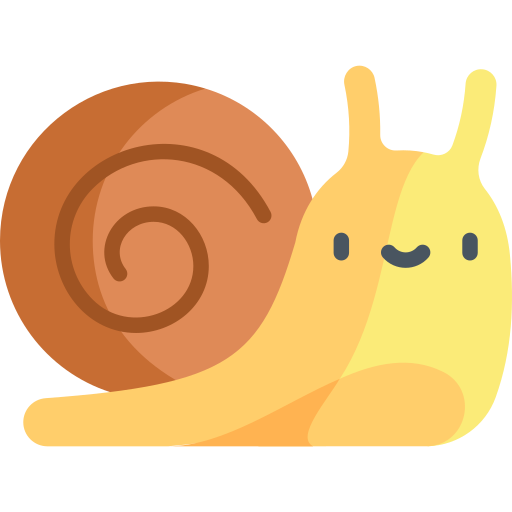
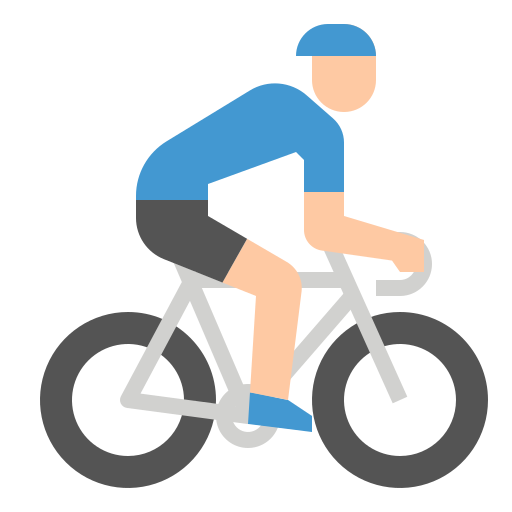
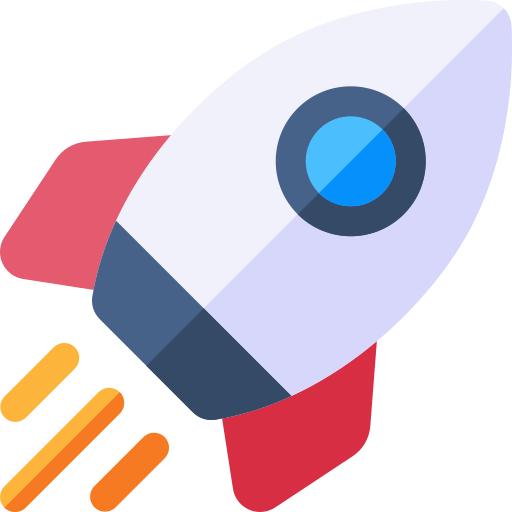